RNAs Involved in Protein Synthesis: Fundamentals and Current Applications
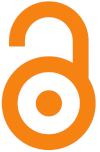
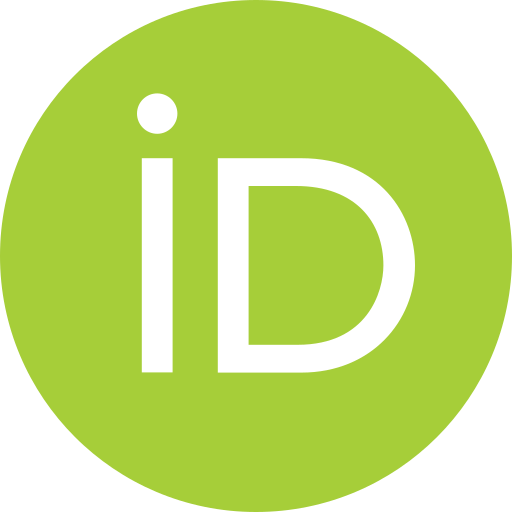
Authors
DOI:
https://doi.org/10.37980/im.journal.ggcl.20232238Keywords:
RNA, gene expression, transcription, translationAbstract
This paper focuses on the diversity of functions and types of RNA, a crucial molecule in cell biology and genetics. RNAs are divided into several categories according to their function and structure. Messenger RNA (mRNA) mediates protein synthesis by copying genetic information from DNA and carrying it to ribosomes. Ribosomal RNAs (rRNAs) are part of ribosomes and are essential for protein synthesis. Transfer RNAs (tRNAs) carry amino acids for protein synthesis. In addition to these, there are RNAs with catalytic activity called ribozymes, which are involved in various chemical reactions, such as RNA splicing and cleavage. Regulatory RNAs, such as microRNAs and interfering RNAs, modulate gene expression and have applications in gene research and gene therapy. Antisense RNAs inhibit specific genes, and long-stranded non-coding RNAs are involved in biological processes such as genetic imprinting and gene regulation in eukaryotes.
Within the last points we wish to highlight the importance of RNAs in biology and their implication in diseases, such as cancer. The possible therapeutic applications of some RNAs, such as the use of RNA interference to treat viral diseases, are also mentioned.
INTRODUCTION
RNA or ribonucleic acid is a molecule composed of sequences of nucleotides linked by phosphodiester bonds. The nucleotides consist of a nitrogenous base, which can be adenine, cytosine, guanine and uracil replacing the thymine of DNA, and a sugar (a ribose). RNA is produced in the nucleus, and it is the DNA that serves as a template for the synthesis of new RNA strands through the process of gene expression, known as transcription. However, many RNAs with the name non-coding RNA (do not encode proteins), originate from self genes (RNA genes), or are the introns rejected during the cutting and splicing process.
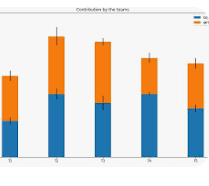
Within the ribonucleic acid family, each RNA has a different function, and it has been chosen to separate them into three major groups: The best known, RNAs involved in protein synthesis, with catalytic activity and regulators (Table 1).
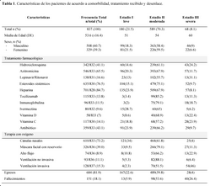
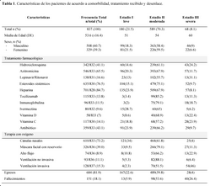
In this review we will mention each of the RNAs in the eukaryotic cell, and emphasize their main functions.
METHODOLOGY
Initially, a search by terms was performed in PubMed and Google Scholar for articles related to RNAs and their functions, using associated terms such as: "types of RNA", "coding RNA", "non-coding RNA", "RNA functions", among others. In addition, databases of scientific journals and specialized books on the subject were consulted to obtain additional information. The search was not limited to a specific year of publication. The selection of articles was based on clearly defined inclusion and exclusion criteria to ensure the relevance and quality of the selected studies. Inclusion criteria were established as follows:
Articles had to address topics related to RNAs and their functions, either in experimental research, literature review or theoretical discussion.
Articles were accepted in several languages to ensure the inclusion of globally relevant research.
Articles from peer-reviewed scientific journals, academic theses and specialized books that met the defined thematic criteria were included.
Exclusion criteria were applied as follows:
Articles that were not related to RNAs and their functions were immediately excluded during the review of titles and abstracts.
Studies that were not available in full text or did not provide relevant information were excluded.
Duplicates and multiple versions of the same study were excluded to avoid redundancy.
RNAs INVOLVED IN PROTEIN SYNTHESIS: FUNDAMENTALS AND CURRENT APPLICATIONS
Messenger RNA
Messenger RNA generally contains a linear single-stranded structure, which can form hairpins at certain stretches whose bases are complementary [5]. In eukaryotes at the 5'end it has a methyl-guanosine group attached to the triphosphate, and at the 3'end it has a polyadenylated (poly-A) tail that stabilizes its half-life, which averages 5 minutes [5].
In eukaryotes, mRNA is synthesized in the nucleoplasm of the cell nucleus from DNA, where it is processed prior to its access to the cytosol.
As mentioned in the previous paragraph, mRNA is the result of a transcription process and its function is to transmit the information contained in DNA to be carried out of the nucleus, associating with ribosomes where the genetic information will be converted into proteins (translation). This is why it is considered an intermediate molecule between DNA and protein and therefore a messenger. In addition to its role in protein synthesis, mRNA is also involved in gene regulation. In this regard, it has been found that the stability and translation efficiency of mRNA can be controlled by mRNA-binding factors through microRNAs, which act as negative regulators of gene expression by binding to mRNA and preventing its translation. Regulation of mRNA is essential for the proper development and function of organisms, and its dysfunction has been linked to various diseases, such as cancer and neurodegenerative diseases [6].
In addition to functioning as a message for any encodable gene, it has been successfully used in genetic engineering in the development of mRNA vaccines, which have the advantage that they can be used to induce the production of any protein by our own cells. For example, during the SARS-CoV-2 pandemic period, Pfizer and Moderna vaccines used messenger RNA coding for the S protein present on the surface of the coronavirus [7], thus allowing the production of the peptide in each patient and mounting an immune response (Figure 2).
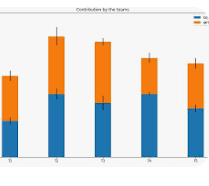
Ribosomal RNA
Ribosomal RNA (rRNA) is a long, single-stranded molecule, which presents fragments with a double-stranded structure in certain regions through folds on itself [8]. These RNAs have an essentially structural function since they associate with proteins to form ribosomes, organelles in charge of protein synthesis.
rRNA is very abundant and represents 80% of the RNA found in the cytoplasm of eukaryotic cells [7], and is also associated with proteins to form ribosomes (the site of protein synthesis).
Ribosomes are divided into large and small subunits. The small subunit contains a single ribosomal RNA molecule, while the large subunit contains one larger and two smaller molecules in eukaryotes. The smallest ribosomal RNA in bacteria can have from 1,500 to 3,000 nucleotides. In humans, ribosomal RNA reaches longer lengths, between 1,800 and 5,000 nucleotides [9].
Ribosomal RNA types are usually classified depending on their displacement during sedimentation, determined by the Svedberg unit [9].
In eukaryotes, 18S ribosomal RNA is found in the small subunit, and the large subunit, 60S, contains three types of ribosomal RNA: 5S, 8S and 28S [9]. Interestingly, these molecules contain enzymatic properties together with other proteins of the ribosome complex [10], thus allowing protein synthesis to take place, being the catalytic component of ribosomes, responsible for creating the peptide bonds between the amino acids of the polypeptide in formation during protein synthesis. The main function of ribosomal RNA is to provide a physical structure that allows taking the messenger RNA and decoding it into amino acids to form proteins [10].
Importantly, ribosomal RNA also plays an important role in the regulation of protein synthesis. The expression of genes coding for rRNA has been found to be regulated by specific transcription factors and regulatory proteins, indicating that the quantity and quality of ribosomes can be finely tuned in response to cellular needs [11]. Furthermore, it has been found that ribosomal RNA can interact with non-coding RNA molecules, such as microRNAs, to regulate protein synthesis in various cellular processes, including cell differentiation and response to stress signals [11].
Transfer RNA
Transfer RNA (tRNA) is an RNA that presents fragments with a double helix structure and others in which "loops" are formed, and is responsible for transporting amino acids in the cytoplasm for protein synthesis. It consists of a range of 70 to 90 nucleotides [12].
The tRNA is clover-shaped and each molecule has two important areas: a trinucleotide region called anticodon and a region where a specific amino acid binds (3' end) [5]. These tRNAs, like other types of RNA, can be post-transcriptionally modified by enzymes. The modification of some of its bases is crucial for mRNA decoding and to maintain the three-dimensional structure of the tRNA.
Transfer RNA (tRNA) plays an essential role in amino acid transport during protein synthesis. Despite its importance, the diversity in tRNA sequence is remarkable, resulting in a wide variety of different tRNAs, each highly specialized in the transport of a specific amino acid; this specialization is based on the nucleotide sequence in the anticodon, which is complementary to the codons present in the messenger RNA (mRNA). However, it is interesting to note that, on occasion, several tRNAs can carry the same amino acid, which might appear redundant at first glance. The apparent redundancy in the ability of tRNAs to carry the same amino acid is related to an evolutionary adaptation that confers robustness to the process of gene translation. This redundancy allows the cell to maintain a constant level of amino acid availability during protein synthesis, even under changing or challenging conditions. For example, in situations of cellular stress or in response to alterations in the environment, some tRNAs can be differentially regulated to ensure the continued production of essential proteins [13].
In more complex organisms, such as humans, about 50 different types of tRNA have been identified, which collaborate to carry the 20 amino acids required for protein synthesis. This apparent redundancy not only supports the stability and accuracy of the translation process, but also allows for greater flexibility and adaptability in cellular response to different environmental conditions and regulatory signals [14].
Additionally, it is worth mentioning that the genetic code is based on the relationship between the anticodon of the tRNA and the amino acid it carries. The tRNA anticodon is the sequence complementary to the codon of the messenger RNA (mRNA), which ensures that the correct amino acid is placed on the polypeptide chain during protein synthesis. This precise interaction between the anticodon and the amino acid is critical for fidelity and specificity in gene translation [15].
On the other hand, tRNA has also been shown to have additional functions beyond its role in protein synthesis. It has been found that certain tRNAs can act as cellular signals and regulate gene expression in response to changes in environmental conditions or cellular stress [16].
tRNAs WITH CATALYTIC ACTIVITY
Ribozymes
The word is the result of unifying "ribonucleic acid" (RNA) with "enzyme" [5].
Their molecular structure presents folds giving rise to three-dimensional structures that form active sites as deep, protected, solvent-inaccessible clefts [5].
Five types of ribozymes are known, three of them carry out self-modification reactions, such as intron deletion or self-cutting, while the others (ribonuclease P and ribosomal RNA) act on different substrates in the catalytic reaction [17].
Their characteristics include accelerating phosphoryl group transfer reactions in orders of magnitude from 10 5 to 10 11 and participating in other reactions, such as splicing; phosphate transesterification, thus participating in the removal of introns from RNA molecules, whether messenger, transfer or ribosomal [17].
Mentioning some of the functional implications of ribozymes, it is possible to modify these molecules, thus representing a powerful biotechnological tool to degrade specific RNAs (gene silencing). Ribozymes, acting as "molecular scissors", will allow RNA to be easily manipulated. For example, one could protect against viruses, bacteria or pathogenic fungi by specifically eliminating their RNA. The possibility of using ribozymes against HIV virus, herpetic viruses and tobacco mosaic virus is under study [18].
Small Nucleolar RNA (snRNA)
So called because of its small size and because it is found in the nucleus of eukaryotic cells and has a single-stranded structure. It is also called U-RNA because of its high uracil content.
They have evolutionarily conserved secondary structures. snRNAs are products of RNA polymerase II transcription; they have a cap in their 5' region, which is hypermethylated [5].
It has been found that snRNAs also play an important role in the RNA splicing process, which is the removal of introns and the joining of exons during precursor RNA maturation. SnRNAs associate with proteins to form small ribonucleoprotein complexes (snRNPs), which bind to splicing sequences in the precursor RNA and catalyze the splicing reaction. Five major types of snRNPs have been identified, each with a different snRNA and a specific set of associated proteins [19]. In addition, snRNAs have also been implicated in other RNA modification processes, such as methylation and pseudouridinylation. Methylation of RNA nucleotides is carried out by specific enzymes and is important for the regulation of translation and RNA stability. Pseudouridinylation is a modification that converts uridine to a ribose-like base, which can affect RNA structure and function [20].
ARN REGULATORS
Interfering RNA
Interfering RNAs (RNAi) are small molecules (20 to 25 nucleotides) that are generated by fragmentation of longer precursors. They can be classified into three major groups: microRNAs, small interfering RNAs and Piwi-associated RNAs, which will be discussed in more detail [21].
It is an RNA molecule that suppresses the expression of specific genes by mechanisms known globally as ribointerference or RNA interference.
RNA interfering RNAs, in addition to their role in suppressing the expression of specific genes, also have an important function in the regulation of cellular processes, such as cell differentiation, apoptosis and immune response [22]. micro-RNAs, for example, have been associated with different types of cancer and neurodegenerative diseases, and have been proposed as potential therapeutic targets [23].
In addition, the use of RNA interference as a therapeutic tool has generated great interest in medicine, especially in the treatment of viral diseases such as HIV and hepatitis B and C [24]. However, it still faces the challenge of overcoming barriers for its efficient delivery to specific cells and tissues [25].
RNA interference (RNAi) is a biological mechanism, widely distributed in eukaryotes, by which, gene silencing is achieved by double-stranded RNA molecules [26].
Small interfering RNA
These are perfectly complementary double-stranded RNA molecules of approximately 20 or 21 nucleotides with 2 unpaired nucleotides at each 3' end. Each RNA strand has a 5' phosphate group and a 3' hydroxyl (-OH) group [27].
Small interfering RNA (siRNA) has been the subject of numerous investigations due to its crucial role in gene regulation and antiviral defense in eukaryotes. Although their mechanism of action is well understood, the various pathways of endogenous and exogenous siRNA production and processing, as well as their relationship with other RNA interference systems, are still being explored [28].
They are frequently produced by viral RNA cleavage, but can also be of endogenous origin. After transcription they are assembled into a protein complex called RISC (RNA-induced silencing complex) that identifies the complementary mRNA which is cleaved into two halves that are degraded by the cellular machinery, thus blocking gene expression [28,29].
It has been shown that siRNAs can be used as tools for genetic research and the development of gene therapies, including targeted regulation of gene expression and suppression of diseases related to abnormal gene expression [7, 18]. These advances have led to a greater understanding of siRNA biology and its potential application in personalized medicine.
micro-RNA
These are short chains of 21 or 22 nucleotides found in eukaryotic cells that are generated from specific precursors encoded in the genome [5].
In animals microRNAs are found encoded in individual genes, grouped in clusters (groups of genes coding for several distinct microRNAs) or in introns of protein-coding genes [18].
These RNAs can modulate the expression of up to 60% of protein-coding genes and are involved in processes such as differentiation, proliferation, apoptosis and development. Therefore, deregulation of processes involving microRNAs can cause various pathologies. Some examples are diabetes, muscular dystrophy, some allergies and cancer [29].
Focusing this RNA at the clinical level, we can say that in humans there are so far between 1,200 and 1,500 micro-RNAs described, which are estimated to be regulating up to one third of the genes of the organism. A given micro-RNA can have several target genes and, in turn, each gene can be the target of several micro-RNAs, forming highly complex regulatory circuits. Its characteristic allows it to currently participate in studies related to the modulation of mRNA translation, thus regulating cholesterol, triglycerides, and energy homeostasis in the organism; micro-RNAs can also function as tumor suppressors or oncogenes; their specific influence on each type of cancer remains to be demonstrated. In fact, a study showed that about 50 % of micro-RNAs annotated in humans are located in areas of the genome known as fragile sites, which are associated with cancer development [30].
One of the features of micro-RNAs that make them so interesting is the possibility of highly specific modification of their expression, which makes them a promising therapeutic tool. Numerous preclinical models have shown that overexpression or inhibition of micro-RNAs can alter pathological responses in cardiovascular diseases [31].
PIWI-associated RNA
Piwi-associated RNAs (Piwi-RNAs or piRNAs) are a third class of interfering RNAs that prevent the expansion of selfish genetic elements (transposons)[32]. This group of molecules has been the last to be identified, and is therefore the least known, although its study is introducing new insights into the understanding of RNA interference mechanisms.
Piwi-associated RNAs are strands of 29-30 nucleotides, specific to animals; they are generated from long single-stranded precursors, in a process that is independent of Drosha (class 2 ribonuclease III enzyme) and Dicer (ribonuclease III family member).
Its main function is the silencing of transposons, however, its lack of control has been found to be associated with the development of various types of cancer. Several piRNAs have been proposed as biomarkers of tumor development, however, not in all types of cancer they have been studied, with breast and gastric cancer leading the list with the highest number of publications [33].
Antisense RNA
Antisense RNA is the complementary, hence non-coding, strand to another mRNA strand. Most inhibit genes, but a few activate transcription. Antisense RNA pairs with its complementary mRNA to form a double-stranded molecule that cannot be translated and is enzymatically degraded [34].
Small pieces of RNA that bind to specific RNA molecules and prevent the RNA from being used to make a protein or alter other cell functions. The use of antisense RNA has been shown to be a promising tool in the study of gene function and gene therapy. In gene therapy, antisense RNA can be designed to inhibit the expression of pathogenic or defective disease-causing genes. For example, the drug nusinersen uses antisense RNA to treat spinal muscular atrophy, a rare genetic disease that affects muscles and mobility [35]. In addition, antisense RNA has been investigated for use in modulating the immune response and preventing the replication of viruses such as HIV. It is also called negative sense RNA and antisense RNA [36].
Long-stranded non-coding RNA
Long-stranded non-coding RNA (lncRNA) molecules constitute a group of genomic transcripts longer than two hundred nucleotides that do not code for proteins [37].
NclRNAs are a heterogeneous group of RNA molecules involved in many biological processes, including sex chromosome inhibition, gene imprinting, and regulation of gene expression in eukaryotes. Currently, nclRNAs are the least understood group of genomic transcripts [37].
RNA production
RNAs are produced in various regions of the cell. The nucleolus, a structure within the nucleus, is the main site of RNA production. Here, RNA polymerase, a crucial enzyme, synthesizes new RNA strands. However, RNA synthesis also occurs in other parts of the nucleus and in the cytoplasm [13].
For example, messenger RNA is formed in the nucleus and then passes into the cytoplasm, where it is translated into proteins by ribosomes. Ribosomal RNA is part of ribosomes, which may be free in the cytoplasm or bound to the endoplasmic reticulum. Transfer RNA is scattered throughout the cytoplasm and brings amino acids to the ribosome.
In eukaryotic cells, RNA synthesis and protein synthesis machinery are separated, allowing more precise control over these processes. This spatial and functional separation allows finer regulation of gene expression and protein synthesis.
DISCUSSION
There are several types of RNA, the three best known and most commonly studied are messenger RNA (mRNA), transfer RNA (tRNA), and ribosomal RNA (rRNA), which are present in all organisms and belong to the group related to protein synthesis [5]; Others have complex regulatory functions in cells, and here we mention interfering RNA, micro-RNA, piwi-associated RNA, antisense RNA and long-chain non-coding RNA, but we also have some involved in catalytic activities; small nucleolar RNA and Ribozymes.
Because of their involvement in many regulatory processes, their abundance and diverse functions, RNAs play an important role in both normal cellular processes and disease.
Important connections between RNA and human diseases have been discovered. For example, it has been described how some microRNAs are able to regulate cancer-associated genes in ways that facilitate tumor development; furthermore, dysregulation of their metabolism has been linked to various neurodegenerative diseases, and other types of RNAs have been linked to the origin of malignancies; however, there are also those that could be used to fight cancer. For example, antisense RNA can be used to block the production of proteins necessary for cell growth and thus stop the replication of malignant cells.
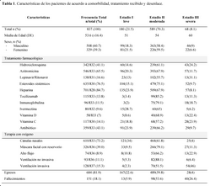
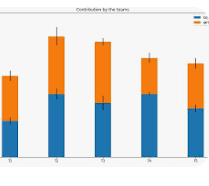
CONCLUSIONS
RNA is a nucleic acid that is composed of three major elements: a nitrogenous base, a five-carbon sugar, and a phosphate group.
RNA molecules are produced in the nucleus of cells, plus they can be found in the cytoplasm. The three main types of RNA molecules are messenger RNA, transfer RNA and ribosomal RNA.
Messenger RNA (mRNA) is the type of RNA that carries information from DNA to ribosomes, where proteins are synthesized. The nucleotide sequence of the mRNA determines the amino acid sequence of the protein. Therefore, it is called coding RNA.
However, many RNAs do not encode proteins, and are called non-coding RNAs; they originate from their own genes or are the introns rejected during the cutting and splicing process (a process that takes place in the cell nucleus and consists of removing the introns, non-coding segments, from the precursor RNA and joining the exons, coding segments, to form the mature messenger RNA). Non-coding RNAs are transfer RNA (tRNA) and ribosomal RNA (rRNA), which are key elements in the translation process, and various types of regulatory RNAs.
Certain non-coding RNAs, called ribozymes, are capable of catalyzing chemical reactions such as cutting and binding other RNA molecules, or forming peptide bonds between amino acids in the ribosome during protein synthesis.
We expect that, in a couple of years, more discoveries will be made in the interest of finding additional links between RNA and disease, as it is likely that a better understanding of RNA and its functions, combined with the continued development of technologies, will reveal new therapeutics for different diseases.
References
Encyclopedia of Genes, Science Direct, Heterogeneous Nuclear RNA, 2001. URL: https://www.sciencedirect.com/topics/medicine-and-dentistry/heterogeneous-nuclear-rna
Dogini DB, Pascoal VDB, Avansini SH, Vieira AS, Pereira TC, Lopes-Cendes I. The new world of RNAs. Genet Mol Biol [Internet]. 2014;37(1):285–93. Available from: https://doi.org/10.1590/S1415-47572014000200014
Donald D. Rao, John S. Vorhies, Neil Senzer, John Nemunaitis; siRNA vs. shRNA: Similarities and differences,Advanced Drug Delivery Reviews,Volume 61, Issue 9,2009, Pages 746-759. URL: https://doi.org/10.1016/j.addr.2009.04.004.
Li, Y. (22 de agosto de 2022). El largo camino hacia el éxito: perfeccionar la próxima generación de vacunas de ARNm. CAS. Recuperado el 25 de septiembre de 2023, de https://www.cas.org/es-es/resources/cas-insights/biotechnology/long-road-success-refining-next-generation-mrna-vaccines
Cango Darwin. (2021). Tipos de ARN con sus funciones, estructuras y conformaciones. Recuperado el 8 de abril de 2023, de studocu Sitio web: https://www.studocu.com/ec/document/universidad-estatal-amazonica/biologia-molecular-ii/tipos-de-arn/13957986
Bartel DP. MicroRNAs: target recognition and regulatory functions. Cell. 2009 Jan 23;136(2):215-33. doi: 10.1016/j.cell.2009.01.002. PMID: 19167326.
Kampers T, Friedhoff P, Biernat J, Mandelkow EM, Mandelkow E. (1996). RNA stimulates aggregation of microtubule-associated protein tau into Alzheimer-like paired helical filaments. FEBS Lett. 399(3), 344-9. doi: 10.1016/s0014-5793(96)01386-5.
Lifeder. (28 de mayo de 2022). ARN ribosomal. Recuperado de: https://www.lifeder.com/arn-ribosomal/.
Gelambi, Mariana. (27 de mayo de 2022). ARN ribosomal. Lifeder. Recuperado de: https://www.lifeder.com/arn-ribosomal/.
Curtis, H., & Schnek, A. (2015). Invitación a la Biología. Ed. Médica Panamericana.
Henras AK, Plisson-Chastang C, O'Donohue MF, Chakraborty A, Gleizes PE. An overview of pre-ribosomal RNA processing in eukaryotes. Wiley Interdiscip Rev RNA. 2015 May-Jun;6(3):225-42. doi: 10.1002/wrna.1277. Epub 2015 Mar 4. PMID: 25737262.
Enrique Cataños. (2015). Estructura y tipos de ARN. Cienciadelux.
Khan Academy. (2023). Los ARNt y los ribosomas. Recuperado el 25 de septiembre de 2023, de Khan Academy
Sampson, J. R., & Uhlenbeck, O. C. (1988). Biochemical and physical characterization of an unmodified yeast phenylalanine transfer RNA transcribed in vitro. Proceedings of the National Academy of Sciences, 85(4), 1033-1037.
National Human Genome Research Institute. (2023). ARN de transferencia (ARNt). Recuperado el 25 de septiembre de 2023, de National Human Genome Research Institute.
Goodarzi, H., Liu, X., Nguyen, H. C., Zhang, S., Fish, L., & Tavazoie, S. F. (2015). Endogenous tRNA-derived fragments suppress breast cancer progression via YBX1 displacement. Cell, 161(4), 790-802.
Lifeder (27 de mayo de 2022). Ribozimas. Lifeder. Recuperado de https://www.lifeder.com/ribozimas/
Carrasco, L. y Almendral del Río, J. (2006). Virus patógenos. Madrid: Editorial Hélice.
Jimenez Ruiz, A. (s.f.). Tema 10-4. Biomolq. Recuperado de https://biomolq.web.uah.es/BM/Esquemas/Tema%2014%20expandido/Temario/Tema%2010-4.htm
Girardot, M., Cavaillé, J., & Feil, R. (2012). Small regulatory RNAs controlled by genomic imprinting and their contribution to human disease. Epigenetics, 7(12), 1341-1348. doi: 10.4161/epi.22884
Siomi H, Siomi MC. On the road to reading the RNA-interference code. Nature. 2009;457(7228):396-404. doi: 10.1038/nature07754
Bartel, D. P. (2004). MicroRNAs: Genomics, biogenesis, mechanism, and function. Cell, 116(2), 281-297.
Kim, V. N., Han, J., & Siomi, M. C. (2009). Biogenesis of small RNAs in animals. Nature Reviews Molecular Cell Biology, 10(2), 126-139.
Tomari, Y., & Zamore, P. D. (2005). Perspective: machines for RNAi. Genes & Development, 19(5), 517-529.
Juliano, R. L. (2016). The delivery of therapeutic oligonucleotides. Nucleic Acids Research, 44(14), 6518-6548.
Noriega, D., Valencia, A., & Villegas, B. (2016). ARN de interferencia (ARNi): una tecnología novedosa con potencial para el control de insectos plaga. Revista U.D.C.A Actualidad & Divulgación Científica, 19(1), 25–35. https://doi.org/10.31910/rudca.v19.n1.2016.107
Bernstein, E., Caudy, A. A., Hammond, S. M., & Hannon, G. J. (2001). Role for a bidentate ribonuclease in the initiation step of RNA interference. Nature, 409(6818), 363-366.
Sontheimer EJ, Carthew RW. (2005). Silence from within: endogenous siRNAs and miRNAs. Cell, 122(1), 9-12.
Doran, A., & Strauss, E. (2006). RNAi Journal - Issue 5 - RNAi in the nervous system. RNAi Journal, 5. Archived from the original on July 16, 2007. Recuperado el 9 de septiembre de 2022, de https://web.archive.org/web/20070716115053/http://www.libpubmedia.co.uk/RNAiJ-Issues/Issue-5/Doran.htm
Esquela-Kerscher A, Slack FJ. Oncomirs - microRNAs with a role in cancer. Nat Rev Cancer. 2006 Apr;6(4):259-69. doi: 10.1038/nrc1840
Díaz Carrasco, I., Guisado Rasco, A. & Ordoñez Fernández, A. (2016, octubre). ¿Qué son los micro-RNA? ¿Para qué sirven? ¿Qué potenciales beneficios podrían tener en el contexto asistencial? Cardiocore, 51(4), 161-166. https://doi.org/10.1016/j.carcor.2015.02.002
Hartig, J. V., Tomari, Y., & Förstemann, K. (2007). piRNAs—the ancient hunters of genome invaders. Genes & Development, 21(14), 1707-1713. https://doi.org/10.1101/gad.1567007
Pérez-Alvarado, Joaquín, & Moreno-Ortiz, José Miguel. (2017). piRNAs, un nuevo campo de biomarcadores en cáncer. Revista biomédica, 28(2), 99-104.
Gilbert, S. F. (2003). Developmental Biology (7th edición). Sinauer. pp. 101
FDA. (2016). FDA approves first drug for spinal muscular atrophy. Consultado el 7 de abril de 2023, de https://www.fda.gov/news-events/press-announcements/fda-approves-first-drug-spinal-muscular-atrophy
Instituto Nacional del Cáncer. (2022). Diccionario de cáncer del NCI. https://www.cancer.gov/espanol/publicaciones/diccionarios/diccionario-cancer/def/arn-de-antisentido
CORDIS. (2022). Function and evolution of long noncoding RNAs. https://cordis.europa.eu/article/id/190859-function-and-evolution-of-long-noncoding-rnas/es
suscripcion
issnes
eISSN L 3072-9610 (English)