Characterization of new genes involved in prostate cancer metastasis.
[Caracterización de nuevos genes implicados en metástasis en cáncer de próstata]Bary Bigay Mercedes1
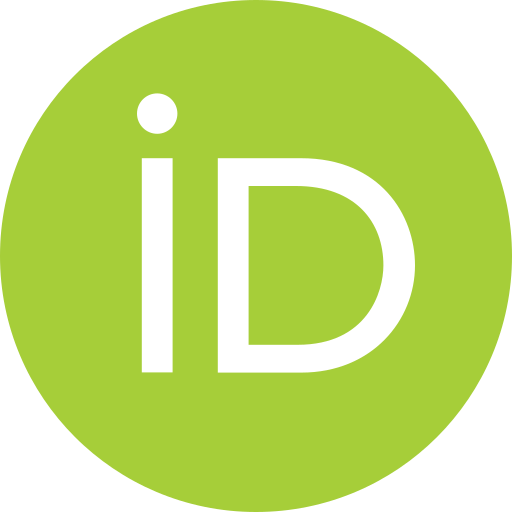
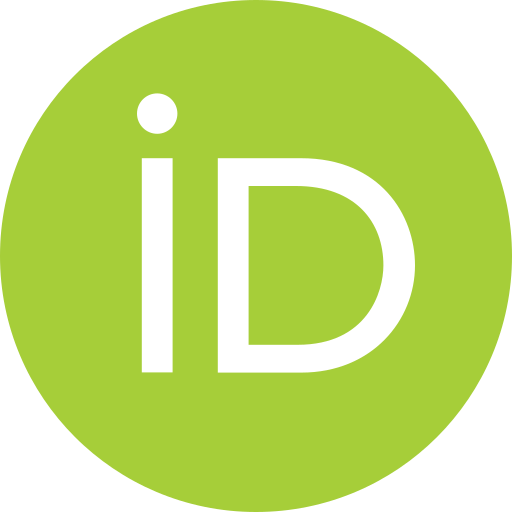
1. ChromoMed Institute, Santo Domingo, Dominican Republic;
Downloads
Abstract
Introduction: Our work addresses a literature review conducted between January 2019 and September 2023, the importance of BRCA1, BRCA2, AR and PTEN genes in the pathogenesis, prognosis and treatment of prostate cancer, especially in its metastatic castration-resistant form (mCRPC), is highlighted. BRCA1 and BRCA2 genes are identified as key markers for predicting cancer aggressiveness, suggesting the need for targeted therapies and strict surveillance. The adaptability of cancer cells and variability in androgen receptor (AR) expression limit the effectiveness of therapies focused solely on AR, pointing to the importance of identifying alternative pathways and biomarkers for more effective treatment. PTEN function is directly related to disease progression, and its alteration suggests potential therapeutic approaches. However, the heterogeneity of cancer cells and complexity of molecular pathways present significant challenges to the development of universal therapies. Conclusion: The findings promote future research to confirm the applicability of these genes as biomarkers and to develop personalized treatment strategies in prostate cancer.
Introduction
Prostate cancer is one of the most common neoplasms in men around the world, and although in many cases it is slow-growing and not lethal, there are aggressive forms of the disease that can lead to metastasis and, therefore, significantly increase mortality [1]. Understanding the molecular and genetic mechanisms underlying the progression and metastasis of this cancer is crucial for developing more effective treatments and improving the prognosis for affected patients.
Metastasis refers to the spread of cancer cells from their original site to other parts of the body, where they can form new tumors. This process is complex and multifaceted, involving the acquisition of skills that allow tumor cells to invade adjacent tissues, enter the bloodstream or lymphatic system, survive in circulation, adhere and extravasate in new sites, and finally proliferate in those distant sites. Over the past few decades, research in the field of prostate cancer has identified several genes and signaling pathways that are involved in tumor progression and metastasis. However, given the heterogeneity and complexity of cancer, it is likely that there are still many genes and mechanisms that play fundamental roles in these processes that are unknown.
Identification and characterization of new genes associated with metastasis in prostate cancer can offer valuable insights into cancer biology and, most importantly, can lead to the development of new targeted therapies. These genes can serve as biomarkers for early detection, prognostic prediction, or as therapeutic targets themselves. Genomic and transcriptomic studies, driven by next-generation sequencing techniques and advanced bioinformatics tools, have revolutionized our ability to discover and understand genetic alterations in cancer.
Using these approaches, it is possible to identify genes whose expression or mutation is associated with metastasis, opening the door to more precise and personalized interventions.
The importance of understanding the epidemiology of prostate cancer (PCa) cannot be underestimated, as it is one of the most prevalent types of cancer globally and is responsible for a large number of deaths associated with this disease. This understanding is crucial for developing effective strategies for both primary and secondary prevention.
Among the risk factors that cannot be altered are elements such as advanced age, inherited genetic mutations, race, and family history. On the other hand, there are also modifiable risk factors, such as metabolic syndrome, obesity, and tobacco consumption, although the evidence for these is less solid.
Although various risk factors related to lifestyle, diet, environment, and infections have also been proposed, the strength of the evidence supporting them is generally low. With this in mind, this systematic and updated review aims to provide a comprehensive and current overview of PCa epidemiology. This includes both modifiable and non-modifiable risk factors, as well as recommendations for screening and strategies for optimization.
RISK FACTORS
Non-modifiable risk factors
It is notable that age factor plays a significant role in the development of prostate cancer (PCa), as shown by various statistics and studies. In the United States, recent cancer figures show a clear correlation between advanced age and the incidence of PCa. For example, while the probability of facing a PCa diagnosis is 1.8% for men aged 60-69, this percentage increases to 9.0% for those aged 70 or older. Over a lifetime, the cumulative probability is 12.5%.
Autopsy-based studies add another layer of complexity to this age-PCa relationship. According to data, 40% of men over 60 who have not undergone screening tests have PCa.
This figure increases to 60% for men over 80. It is also relevant to note that, according to the International Society of Urological Pathology, 32% of these post-mortem diagnosed cancers are grade 2 or higher.
Family history
Determining the precise connection between a family history of prostate cancer (PCa) and the likelihood of developing this disease is complex, although it is clear that there is a high risk. This risk not only refers to the development of PCa in general, but could also apply to more severe and deadly forms of the disease. Several elements come into play when evaluating this risk, such as the number of affected family members, the level of relationship with them (whether they are first or second degree relatives), and additional factors such as their age at the time of diagnosis and death due to PCa.
In addition, factors such as the type of cancer that other family members may have had (such as breast or ovarian cancer) or the presence of high-grade disease in the family, can further complicate the risk evaluation. There are variables that can distort this data, such as memory bias and the overall health status of the family. It is relevant to mention that, according to studies, men who have a brother or son with PCa face a risk that is approximately 2.5 times higher of developing the disease. This kind of information is crucial to understand how familial risk factors can contribute to the development of PCa [2].
Genetic predisposition
The genetic heritability in the case of prostate cancer (PCa) is notably high compared to other more common types of cancer. According to research with twins, the heritability of PCa is estimated to be approximately 58%.
Genetic susceptibility manifests itself in two different ways: there are genetic factors that are rare but highly penetrant, and others that are more prevalent but generally less penetrant. For example, mutations in the HOXB13 and BRCA2 genes are the most well-known for their relation to autosomal dominant susceptibility to PCa. However, it is important to keep in mind that these mutations are relatively rare in the general population, with an estimated prevalence of only around 0.3%.
Science is increasingly revealing the connection between prostate cancer (PCa) risk and mutations in various genes that have roles in DNA repair. Among these genes, BRCA1, ATM, CHEK2, NBS1, and those associated with Lynch syndrome (such as MLH1, MSH2, MSH6, and PMS2) stand out as particularly relevant. It is important to note that these mutations have a higher prevalence in cases of advanced PCa, especially in its metastatic form, compared to localized PCa.
In a significant study by the UK Biobank involving more than 21,000 men, it was discovered that 1.4% had a rare pathogenic mutation in at least one of the key genes: BRCA2, HOXB13, and CHEK2. Although these mutations are rare in the general population and in patients with low-risk PCa, their prevalence increases significantly in cases of advanced PCa, reaching figures between 10% and 20%. The variability in the incidence and aggressiveness of the disease due to these genetic mutations shows the complexity of the genetic relationship and the risk of developing PCa. These findings highlight the need for further research to fully understand how these genetic factors contribute to the development and progression of PCa [2].
In the search to understand the correlation between male pattern baldness and prostate cancer (PCa), four significant meta-analyses have been carried out. Interestingly, these studies failed to find a direct link between male pattern baldness as a whole and a higher likelihood of developing PCa. However, subgroup analyses within these studies did yield more specific findings. Specifically, they identified a relationship between baldness in the vertex region, which is the top of the head, and an elevated risk of developing PCa.
This discovery suggests that, although male pattern baldness in general does not seem to be associated with a higher risk of PCa, certain balding patterns, such as those that occur in the vertex area, could be indicative of an increased risk.
High stature has been consistently correlated with an increased probability of developing prostate cancer (PCa), and more specifically, its high-grade variants. One possible mechanism that could explain this association is the presence of elevated levels of insulin-like growth factor during puberty.
This finding suggests that body dimensions, particularly height, could be an important indicator in assessing the risk of PCa. The relationship between height and levels of certain growth factors during critical stages of development could provide valuable clues to better understand the etiology of this disease [2].
Modifiable risk factors!
Although the relationship between sedentary behavior and increased risk of prostate cancer (PCa) has not been clearly established by several studies, it has been observed that a higher level of physical activity can have significant benefits. In particular, individuals who are more physically active have a lower specific mortality rate from PCa and, in the case of diagnosed patients, higher rates of disease-free survival.
This positive effect has been observed in both intense physical activities and brisk walking. Most of the research in this area has focused on recreational physical activity, leaving a gap in knowledge about the potential effects of physical activity in the workplace. However, there are indications that suggest that staying active at work could also reduce the risk of PCa.
On the other hand, regarding sleep, its duration does not seem to have a significant effect on the overall risk of developing prostate cancer (PCa). However, some studies have suggested that the quality of sleep may be related to a higher risk of aggressive forms of the disease. These findings suggest that, beyond the duration of sleep, the quality of sleep could have an impact on the severity of PCa.
Among the different diets evaluated for their impact on prostate cancer (PCa), some dietary patterns show certain promises. The Mediterranean diet, for example, has shown a modest protective association against PCa in individuals who strictly follow the diet, although other studies do not support this relationship. Vegetarian diets also show potential, as studies have confirmed a lower risk of PCa in vegetarians compared to meat consumers.
In the context of plant-based diets, findings are mixed: some studies have found a slight protection against PCa, while others have not established any significant relationship. However, both vegetarian diets and pesco-vegetarian and semi-vegetarian diets are included in this group. It is worth noting that in intervention studies with diets completely based on plants, positive oncological results were observed in the short term in men already diagnosed with PCa [2].
In the context of prostate cancer (PCa), dietary inflammation seems to play a key role. Several studies have investigated the correlation between a diet with a high Dietary Inflammatory Index and the risk of PCa, finding a positive association in all cases. This evidence suggests that a diet that promotes inflammation could be a risk factor for the development of the disease. Furthermore, the combination of a diet that causes both inflammation and hyperinsulinemia could have even more serious implications.
This type of diet has been associated with an increased risk of developing more aggressive forms of PCa. This highlights the importance of considering the inflammatory and hyperinsulinemic nature of the diet when evaluating risk factors for this form of cancer [2].
The relationship between protein intake and the risk of prostate cancer (PCa) appears to be more complex than initially thought. While a direct association between total protein intake and PCa risk was not found, the data suggest that not all proteins are equal in this context.
In particular, dairy protein shows a dose-dependent effect on the risk of developing PCa. For men who consume more than 30 grams of dairy protein per day, the risk of PCa increases. Each additional 20 grams per day of dairy protein is associated with a cumulative relative risk increase of 1.1%. This finding emphasizes the importance of considering the type of proteins consumed, beyond just the total amount, when evaluating risk factors for PCa [2].
The relationship between occupational exposure to certain substances and the risk of prostate cancer (PCa) has been the subject of several studies, but the results are mixed and inconclusive. Two reviews and meta-analyses on exposure to asbestos showed conflicting results: one found no association with the risk of PCa, while the other reported a weak association.
Other work-related factors were also studied, including exposure to persistent, bioaccumulative, and toxic chemical compounds, the broad-spectrum insecticide permethrin, and job-related stress. None of these factors showed a correlation with an increased risk of PCa. However, a possible positive association was identified with occupational exposure to cobalt and hexavalent chromium.
A higher likelihood of developing prostate cancer was observed in two specific occupational groups: firefighters and police officers. It is important to note, however, that the results were heterogeneous among the different studies considered, and it is possible that a higher rate of PSA testing in these populations may have influenced the results obtained.
In addition, it was found that workers with chemical and pesticide exposures in the workplace, such as agricultural and oil workers, faced an increased risk of prostate cancer. However, in relation to occupational physical effort, a clear association with a higher risk of PCa was not identified.
Primary prevention/chemoprevention
5-alpha reductase inhibitors have been studied in high-quality randomized trials to evaluate their effect on the risk of prostate cancer. Comprehensive studies have investigated whether these medications can reduce the risk of prostate cancer.
The results indicate that after several years, men who took these medications were less likely to develop prostate cancer compared to those who received an inactive placebo. This suggests that 5-alpha reductase inhibitors could play an important role in the prevention of this disease.
Anti-inflammatories. Regular and continuous use of aspirin has been linked to a lower risk of developing prostate cancer (PCa). Studies have shown that consistent use of aspirin significantly reduced the risk of PCa [2].
Statins. The effect of statin use on the occurrence of prostate cancer (PCa) is still a topic of debate, but evidence suggests that statins could decrease the risk of aggressive PCa and, among diagnosed patients, reduce mortality rates [2].
Vitamins and supplements. It has been confirmed that vitamin D does not affect the overall incidence of prostate cancer (PCa). However, it was observed that higher levels of 25-hydroxyvitamin D in the bloodstream are related to an increased risk of PCa, showing a non-linear dose response [2].
Secondary prevention (screening and early detection)
In December 2022, the Council of the European Union recommended member countries to consider the possibility of establishing organized prostate cancer screening programs, using prostate-specific antigen (PSA) tests and magnetic resonance imaging (MRI) studies [3].
The guidelines issued in 2021 by EAU-EANM-ESTRO-ESUR-SIOG also support the idea of providing prostate-specific antigen (PSA) tests to men who are properly informed. However, these guidelines suggest considering the assessment and possible discontinuation of tests based on life expectancy and overall health status of individuals [4].
In the United States, the guidelines issued in 2018 by the US Preventive Services Task Force state that men aged 55 to 69 should make personalized decisions about undergoing periodic screening exams based on prostate-specific antigen (PSA) for prostate cancer [5].
The PROMIS study demonstrated that the implementation of multiparametric magnetic resonance imaging (mpMRI) prior to biopsy could reduce the number of initial biopsies by 27% and lead to 5% fewer detections of clinically insignificant cancers compared to standard transrectal ultrasound (TRUS) biopsy. In turn, the PRECISION study supports the utility of mpMRI, as 28% of men in the MRI group had a normal MRI and were able to avoid the need for biopsy [6].
Genetic mutations are key elements in the development of cancer, with this disease being considered of genetic origin. These mutations can be divided into two main categories: inactivating mutations, which affect tumor suppressor genes, and activating mutations, which impact oncogenes.
Regarding inactivating mutations, these occur due to structural reorganizations in the genome, which can result in the loss of DNA segments through deletions, whether extensive or focused. These changes can affect one or both copies of a gene, generating monoallelic or biallelic mutations.
On the other hand, activating mutations can originate through different mechanisms, such as amplifying the number of copies of a gene, point mutations affecting individual bases of DNA, or structural reorganizations leading to gene fusion.
In the context of prostate cancer, although the complexity of its karyotype has complicated its study, significant chromosomal alterations were identified through cytogenetic studies in the 1980s. These alterations involve specific regions such as 8p, 8q (C-MYC locus), 10p (PTEN locus), 17q (TP53 locus), and the androgen receptor (AR).
Technological advances, such as polymorphic fragment length analysis and fluorescence in situ hybridization (FISH), have allowed for a more detailed analysis of recurrent chromosomal alterations in prostate cancer.
Advancements in high-throughput technologies, such as next-generation sequencing (NGS), have provided an extraordinary insight into the genome/transcriptome of PCa. In recent genomic studies based on NGS, the presence of many known genomic events in PCa has mostly been confirmed, such as loss of PTEN, mutation/loss of TP53. An important observation is that certain events, such as SPOP mutation, appear as the most common point mutation in primary PCa [7].
The presence or absence of recurrent gene fusions between the androgen-regulated gene TMPRSS2 and members of the ETS family (most commonly ERG). The complete profile of copy number, whole exome sequencing (WES), and whole genome sequencing (WGS) have allowed to identify some genes mutated somatically with high recurrence including SPOP, TP53, PTEN, and FOXA1.
These computational evaluations, performed on a wide collection of prostatectomy samples characterized by WES or WGS, have deepened our understanding of early events versus later events in prostate carcinogenesis [7].
The genomic revolution has fundamentally changed our understanding of prostate cancer, shifting the conversation from a generalized perspective to a much more personalized and precise one.
The identification of genetic markers, such as BRCA2, especially in high-risk populations such as men with metastatic disease, has provided a lens through which more effective and precise treatment strategies can be designed. This genomic revolution has been the driving force behind new guidelines for performing germline DNA testing in prostate cancer patients.
Not only does it provide a more complete picture of the individual's genetic landscape, but it also opens the door to targeted treatments that are increasingly specific and effective.
As more genes associated with the risk of prostate cancer are revealed, a new world of therapeutic possibilities is opening up.
We are already seeing how the Food and Drug Administration of the United States (FDA) has begun to approve specific drugs based on these genetic identifications. These advancements represent a major step towards precision medicine in the treatment of prostate cancer, allowing for a more tailored and effective strategy that can vary significantly from one patient to another.
This era of genomic medicine is not only promising for treatment, but also has significant implications for early detection and prevention. By better understanding the genetic factors that contribute to prostate cancer, we could be much better equipped to carry out effective preventive interventions. In the near future, access to genomic information could become a standard tool in the fight against this disease, forever changing the way we approach it.
In this research, we will focus on discovering and understanding the "New genes" that contribute to the phenomenon of metastasis in prostate cancer. However, since the evidence for certain genes is limited, we will focus our characterization on those genes that have scientific relevance and clinical validity for their use as molecular genetic biomarkers in the study of progression to metastasis in Prostate Cancer.
The purpose of this literature review is to identify new associated genes that could be used for the diagnosis, monitoring, and treatment of this aggressive form of cancer, such as the ones mentioned below:
BRCA1/BRCA2: Although better known for their relationship with breast and ovarian cancer, these genes have also been implicated in advanced prostate cancer.
PTEN: This gene is a tumor suppressor and its loss or mutation has been associated with various types of cancer, including prostate cancer.
AR (Androgen Receptor): Although not a "new" gene, mutations and variants in this gene continue to be an area of intense research, especially in relation to resistance to antiandrogen therapy.
MATERIAL AND METHODS
The methodology did not focus on laboratory experiments or the collection of primary data, but on the collection, analysis, and synthesis of previously published literature on the subject.
Sample Selection
Sources. We conducted a thorough search in databases and academic and scientific search engines, such as PubMed, Scopus, Web of Science, and Google Scholar.
We used keywords and related combinations related to the topic, such as: "prostate cancer", "metastasis", "genes", "genetic characterization", among others.
Study selection
After obtaining an initial set of studies, we proceeded to review titles and abstracts to select those that meet the inclusion criteria. Subsequently, we read the complete studies to ensure their relevance and quality.
Data extraction
We created a format or template for data extraction that contained: author(s), year of publication, study title, identified genes, type of genes, and relevance in metastasis. We extracted relevant information from each selected study and filled in the template.
Analysis techniques
Analysis and synthesis of information. We grouped the studies according to the publication date from the oldest to the most recent, identified genes, and study results. We synthesized the collected information, identifying patterns, similarities, differences, and gaps in the literature.
Validation Methods
In the context of a literature review, validation consisted of ensuring that the study is rigorous and that the results are reliable and reproducible.
Source Validation
We only used reliable academic sources that have been peer-reviewed to ensure that the information we used is valid and reliable.
Cross-Verification of Information
Ensuring that findings are replicated in multiple different studies is a form of validation.
Inclusion and Exclusion Criteria
Inclusion: Studies published from January 2019 to September 2023, articles from peer-reviewed scientific journals, studies addressing the molecular genetics of prostate cancer and metastasis, studies in humans or human tumors, and articles in English.
Exclusion: Studies prior to this period, studies not peer-reviewed, research not focused on molecular genetics or not related to prostate cancer, studies in animals, and articles in languages other than English.
RESULTS AND DISCUSSION
Genes BRCA1 and BRCA2
A total of 464 studies have been reviewed, published in PubMed, Scopus, Web of Science, and Google Scholar, from January 2019 to September 2023, related to prostate cancer, of which only 12 met the inclusion criteria. They address the relationship between genetics and prostate cancer, organized chronologically from the most recent to the oldest.
These studies have investigated the presence of mutations in tumor suppressor genes, such as BRCA1 and BRCA2, and their influence on the risk, aggressiveness, and prognosis of prostate cancer.
The most recent studies, carried out in 2023, have highlighted the importance of genetics in risk stratification and the development of targeted therapies in prostate cancer. Mutations in BRCA2 have been observed to be associated with more aggressive disease and high specific mortality from prostate cancer.
Furthermore, a higher frequency of mutations in tumor suppressor genes such as BRCA2 has been found in prostate cancer patients. These studies have underscored the need to consider genetics in the early identification of high-risk patients and in making therapeutic decisions.
The study by Oh M et al. (2019) and Tommy Nyberg et al. (2020) provide a solid baseline for understanding how mutations in BRCA1/2 are associated with increased risk and mortality. Specifically, Nyberg and colleagues emphasize that the location of the mutation within the gene and family history are also crucial factors. This suggests that not all BRCA2 mutations are equal and that genetic and family context could be crucial for interpreting risk.
Hussain M et al. (2020) go further and examine the efficacy of the drug Olaparib in a subset of patients with alterations in BRCA1/2. This study suggests a pathway for personalized therapeutic interventions based on the patient's genetic profile, supporting the importance of early genetic screening to guide therapy.
Junejo NN et al. (2020) and Patel VL et al. (2020) establish a clear link between BRCA2 mutations and a faster progression of the disease, including a shorter survival time and higher grades on the Gleason scale.
The study by Dall'Era MA et al. (2020) expands the focus beyond BRCA1/2, including other DNA repair genes such as ATM, MSH6, and MSH2.
The finding that approximately 16% of a cohort of 944 men had at least one mutation in these genes suggests that attention should not be limited to BRCA1/2 alone.
Wokororczyk D et al. (2020) offer a geographical perspective, suggesting that the prevalence of mutations and their relationship with prostate cancer aggressiveness may vary by population, indicating the need for more diversified studies worldwide.
Stadler ZK et al. (2021) and Valsecchi AA et al. (2023) emphasize the importance of expanding targeted therapy and address the current limitations in the clinical application of these genetic findings. In particular, Valsecchi points out that somatic mutations are more common than germline mutations, an area that requires further research.
These studies demonstrate that mutations in BRCA1/2 and other genes related to DNA repair play a significant role in the aggressiveness and prognosis of prostate cancer. However, the clinical implications of these findings, such as the implementation of personalized therapies and early detection, are still under development.
Furthermore, future research could focus on the impact of somatic mutations versus germline mutations, as well as the geographic and ethnic variability in these mutation patterns.
Gene AR (Androgen Receptor)
In the corpus of 159 articles reviewed in PubMed, Scopus, Web of Science, and Google Scholar, focusing on the androgen receptor (AR), only 10 met the strict inclusion criteria, highlighting the complexity and challenges in the research of metastatic castration-resistant prostate cancer (mCRPC).
These 10 studies are particularly important as they offer robust clinical and molecular findings, specifically focused on how AR and its associated pathways impact the progression and treatment of metastasis in mCRPC.
One key point is the adaptability of cancer cells. Studies indicate that even when AR is inhibited, cancer cells can adapt and find alternative pathways to survive and metastasize. This poses a significant challenge in the clinical management of mCRPC, suggesting that therapies targeting only AR may not be sufficiently effective in preventing or controlling metastasis.
Regarding the implications for metastasis, the plasticity of cancer cells in mCRPC is particularly concerning. Studies show a trend towards heterogeneity in AR expression during treatment, as well as an increase in neuroendocrine marker expression. This could allow cancer cells to more easily colonize new tissues, making the disease harder to treat and more likely to spread. Additionally, alterations in AR and other genes such as TP53, RB1, and PTEN have direct implications on how and where metastasis occurs.
These genetic mutations could serve as biomarkers to predict which patients are at higher risk of developing metastasis and could benefit from more aggressive treatments or new therapeutic modalities. These studies provide valuable knowledge about the role that AR plays in metastasis of mCRPC, and emphasize the need for more complex and personalized therapeutic strategies. By recognizing the adaptability and heterogeneity of cancer cells in mCRPC, as well as the different mutations and pathways that contribute to metastasis, researchers and physicians can move closer to personalized treatment to improve outcomes in this complicated and devastating disease.
PTEN gene
After reviewing 414 scientific articles in PubMed, Scopus, Web of Science, and Google Scholar, associated with the PTEN gene in relation to prostate cancer, only 13 met the inclusion criteria for a detailed analysis. Specifically, the focus of this analysis is on findings related to metastasis, a critical phenomenon that significantly complicates the treatment and prognosis of prostate cancer.
The articles demonstrate that loss of function or mutation in the PTEN gene is closely related to progression towards advanced and metastatic states of cancer. For example, a 2020 study titled "Genomics of lethal prostate cancer at diagnosis and castration resistance" points out that loss of function in genes such as TP53, RB1, and PTEN is more common in cases of metastatic castration-resistant prostate cancer (mCRPC) compared to non-metastatic states of cancer. This finding suggests a critical role of PTEN in the aggressiveness and treatment resistance of advanced cancer.
Another article from 2022, "Prevalence and prognosis of next-generation therapeutic targets in metastatic castration-resistant prostate cancer," expands this understanding by linking the loss of PTEN with shorter progression-free survival in patients treated with abiraterone, a commonly used drug in mCRPC. This study suggests that alterations in PTEN could be used as a biomarker to predict treatment response and potentially as a therapeutic target.
It is clear that PTEN plays a key role not only in the initiation of prostate cancer but also in its progression to metastatic states. These findings raise the possibility that restoring normal PTEN function, or modulating its signaling pathways, may offer new therapeutic strategies in the treatment of metastatic prostate cancer.
Tumor heterogeneity and the complexity of signaling pathways involved in metastasis make the development of effective therapies a challenge. In conclusion, the selected articles offer robust evidence of the importance of the PTEN gene in prostate cancer metastasis, opening new avenues for the development of targeted therapeutic strategies and more effective treatment.
CONCLUSIONS
Our literature review on prostate cancer conducted with scientific articles extracted from PubMed, Scopus, Web of Science, and Google Scholar, between the period of January 2019 to September 2023, has highlighted the critical importance of several genes involved in the pathogenesis, prognosis, and treatment of this disease, particularly in its metastatic castration-resistant form (mCRPC).
BRCA1 and BRCA2 Genes
These genes are key markers in identifying patients who have a higher risk of developing more aggressive forms of prostate cancer.
The presence of mutations in BRCA1 and BRCA2 could open doors to specific targeted therapies, as well as more strict surveillance.
Studies underline that more research is needed to understand the exact role these genes play in prostate cancer and how they can be effectively integrated into treatment strategies.
AR gene (Androgen Receptor)
Cancer cells have the ability to adapt and find alternative ways to survive, making AR-focused therapies insufficient.
Studies have shown variability in AR expression during treatment, and an increase in neuroendocrine marker expression, making disease control more difficult.
Mutations in AR and genes like TP53, RB1, and PTEN could be used to predict disease behavior and be targets for future therapies.
PTEN gene
Role in Disease Progression: There is a close relationship between loss of function or mutations in PTEN and progression towards more advanced and metastatic stages of prostate cancer.
Changes in PTEN may influence the effectiveness of existing treatments such as abiraterone and could be used to guide therapeutic decisions.
Studies suggest that restoring PTEN function or modulating its signaling pathways could offer novel treatment strategies.
The adaptability and heterogeneity of cancer cells, combined with the complexity of the molecular pathways involved, make it difficult to create universally effective therapies.
The results are preliminary and further research is needed to confirm these findings, especially to understand how they can be effectively implemented as clinical biomarkers and at what stage of the disease. The adaptability and heterogeneity of cancer cells, along with the complexity of the molecular pathways involved, pose significant challenges for the development of effective therapies.
Although these findings offer promising directions for personalized treatment of prostate cancer and the utilization of characterized genes for use as biomarkers, further research is needed to validate these results and fully understand their clinical applications.
TABLES
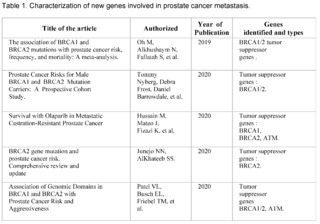
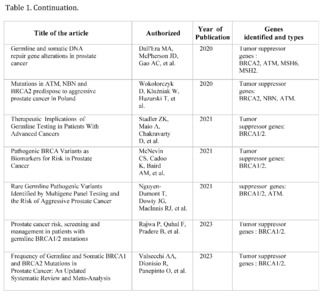
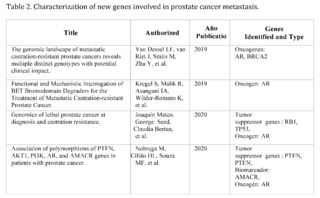
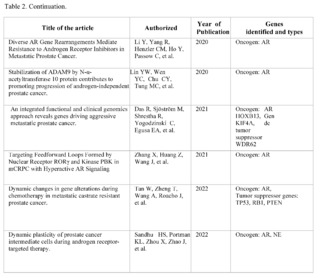
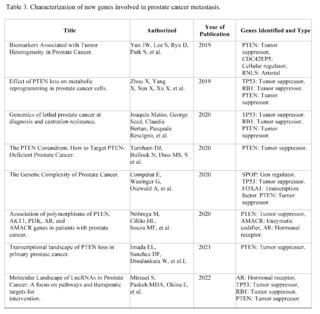
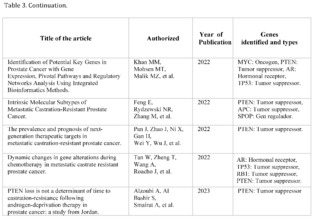
References
[1] Organización Panamericana de la Salud. (2020). Cáncer. Recuperado de https://www.paho.org/es/temas/cancer
[2] Bergengren, O., Pekala, K. R., Matsoukas, K., Fainberg, J., Mungovan, S. F., Bratt, O., Bray, F., Brawley, O., Luckenbaugh, A. N., Mucci, L., Morgan, T. M. y Carlsson, S. V. (2023). Actualización 2022 sobre la epidemiología y factores de riesgo del cáncer de próstata: Una revisión sistemática. European Urology, 84(2), 191-206.
[3] Consejo de la Unión Europea. (2022, 9 de diciembre). El Consejo actualiza su Recomendación para el cribado del cáncer [Comunicado de prensa]. https://data.consilium.europa.eu/doc/document/ST-14770-2022-INIT/es/pdf
[4] Mottet, N., van den Bergh, R. C. N., Briers, E., Van den Broeck, T., Cumberbatch, M. G., De Santis, M., ... & Cornford, P. (2021). EAU-EANM-ESTRO-ESUR-SIOG Guidelines on Prostate Cancer-2020 Update. Part 1: Screening, Diagnosis, and Local Treatment with Curative Intent. European Urology, 79(2), 243-262. https://doi.org/10.1016/j.eururo.2020.09.042
[5] Plambeck, B. D., Wang, L. L., McGirr, S., Jiang, J., Van Leeuwen, B. J., LaGrange, C. A., & Boyle, S. L. (2022). Effects of the 2012 and 2018 US Preventive Services Task Force prostate cancer screening guidelines on pathologic outcomes after prostatectomy. Prostate, 82(2), 216-220. doi: 10.1002/pros.24261.
[6] El-Shater Bosaily, A., Parker, C., Brown, L. C., Gabe, R., Hindley, R. G., Kaplan, R., ... Ahmed, H. U. (2015). PROMIS—Prostate MR imaging study: A paired validating cohort study evaluating the role of multi-parametric MRI in men with clinical suspicion of prostate cancer. Contemporary Clinical Trials, 42, 26-40. https://doi.org/10.1016/j.cct.2015.02.008
[7] Rubin, M. A., & Demichelis, F. (2019). The Genomics of Prostate Cancer: A Historic Perspective. Cold Spring Harbor Perspectives in Medicine, 9(3), a034942. https://doi.org/10.1101/cshperspect.a034942
[8] Oh, M., Alkhushaym, N., Fallatah, S., Althagafi, A., Aljadeed, R., Alsowaida, Y., Jeter, J., Martin, J. R., Babiker, H. M., McBride, A., & Abraham, I. (2019). The association of BRCA1 and BRCA2 mutations with prostate cancer risk, frequency, and mortality: A meta-analysis. Prostate, 79(8), 880-895. https://doi.org/10.1002/pros.23795
[9] Nyberg, T., Frost, D., Barrowdale, D., Evans, D. G., Bancroft, E., Adlard, J., Ahmed, M., Barwell, J., Brady, A. F., Brewer, C., Cook, J., Davidson, R., Donaldson, A., Eason, J., Gregory, H., Henderson, A., Izatt, L., Kennedy, M. J., Miller, C., Morrison, P. J., Murray, A., Ong, K. R., Porteous, M., Pottinger, C., Rogers, M. T., Side, L., Snape, K., Walker, L., Tischkowitz, M., Eeles, R., Easton, D. F., & Antoniou, A. C. (2020). Prostate Cancer Risks for Male BRCA1 and BRCA2 Mutation Carriers: A Prospective Cohort Study. European Urology, 77(1), 24-35. https://doi.org/10.1016/j.eururo.2019.08.025
[10] Hussain, M., Mateo, J., Fizazi, K., Saad, F., Shore, N., Sandhu, S., Chi, K. N., Sartor, O., Agarwal, N., Olmos, D., Thiery-Vuillemin, A., Twardowski, P., Roubaud, G., Özgüroğlu, M., Kang, J., Burgents, J., Gresty, C., Corcoran, C., Adelman, C. A., & de Bono, J.; PROfound Trial Investigators. (2020). Survival with Olaparib in Metastatic Castration-Resistant Prostate Cancer. The New England Journal of Medicine, 383(24), 2345-2357. https://doi.org/10.1056/NEJMoa2022485
[11] Junejo, N. N., & AlKhateeb, S. S. (2020). BRCA2 gene mutation and prostate cancer risk: Comprehensive review and update. Saudi Medical Journal, 41(1), 9-17. https://doi.org/10.15537/smj.2020.1.24759
[12] Patel, V. L., Busch, E. L., Friebel, T. M., Cronin, A., Leslie, G., McGuffog, L., ... & Rebbeck, T. R. (2020). Association of Genomic Domains in BRCA1 and BRCA2 with Prostate Cancer Risk and Aggressiveness. Cancer Research, 80(3), 624-638. https://doi.org/10.1158/0008-5472.CAN-19-1840
[13] Dall'Era, M. A., McPherson, J. D., Gao, A. C., DeVere White, R. W., Gregg, J. P., & Lara, P. N. Jr. (2020). Germline and somatic DNA repair gene alterations in prostate cancer. Cancer, 126(13), 2980-2985. https://doi.org/10.1002/cncr.32908
[14] Wokołorczyk, D., Kluźniak, W., Huzarski, T., Gronwald, J., Szymiczek, A., Rusak, B., Stempa, K., Gliniewicz, K., Kashyap, A., Morawska, S., Dębniak, T., Jakubowska, A., Szwiec, M., Domagała, P., Lubiński, J., Narod, S. A., Akbari, M. R., Cybulski, C., & the Polish Hereditary Prostate Cancer Consortium. (2020). Mutations in ATM, NBN and BRCA2 predispose to aggressive prostate cancer in Poland. International Journal of Cancer, 147(10), 2793-2800. https://doi.org/10.1002/ijc.33272
[15] Stadler, Z. K., Maio, A., Chakravarty, D., Kemel, Y., Sheehan, M., Salo-Mullen, E., Tkachuk, K., Fong, C. J., Nguyen, B., Erakky, A., Cadoo, K., Liu, Y., Carlo, M. I., Latham, A., Zhang, H., Kundra, R., Smith, S., Galle, J., Aghajanian, C., Abu-Rustum, N., Varghese, A., O'Reilly, E. M., Morris, M., Abida, W., Walsh, M., Drilon, A., Jayakumaran, G., Zehir, A., Ladanyi, M., Ceyhan-Birsoy, O., Solit, D. B., Schultz, N., Berger, M. F., Mandelker, D., Diaz, L. A. Jr., Offit, K., & Robson, M. E. (2021). Therapeutic Implications of Germline Testing in Patients With Advanced Cancers. Journal of Clinical Oncology, 39(24), 2698-2709. https://doi.org/10.1200/JCO.20.03661
[16] McNevin, C. S., Cadoo, K., Baird, A. M., Murchan, P., Sheils, O., McDermott, R., & Finn, S. (2021). Pathogenic BRCA Variants as Biomarkers for Risk in Prostate Cancer. Cancers (Basel), 13(22), 5697. https://doi.org/10.3390/cancers13225697
[17] Nguyen-Dumont, T., Dowty, J. G., MacInnis, R. J., Steen, J. A., Riaz, M., Dugué, P. A., Renault, A. L., Hammet, F., Mahmoodi, M., Theys, D., Tsimiklis, H., Severi, G., Bolton, D., Lacaze, P., Sebra, R., Schadt, E., McNeil, J., Giles, G. G., Milne, R. L., & Southey, M. C. (2021). Rare Germline Pathogenic Variants Identified by Multigene Panel Testing and the Risk of Aggressive Prostate Cancer. Cancers (Basel), 13(7), 1495. https://doi.org/10.3390/cancers13071495
[18] Rajwa, P., Quhal, F., Pradere, B., Gandaglia, G., Ploussard, G., Leapman, M. S., Gore, J. L., Paradysz, A., Tilki, D., Merseburger, A. S., Morgan, T. M., Briganti, A., Palapattu, G. S., & Shariat, S. F. (2023). Prostate cancer risk, screening and management in patients with germline BRCA1/2 mutations. Nature Reviews Urology, 20(4), 205-216. https://doi.org/10.1038/s41585-022-00680-4
[19] Valsecchi, A. A., Dionisio, R., Panepinto, O., Paparo, J., Palicelli, A., Vignani, F., & Di Maio, M. (2023). Frequency of Germline and Somatic BRCA1 and BRCA2 Mutations in Prostate Cancer: An Updated Systematic Review and Meta-Analysis. Cancers (Basel), 15(9), 2435. https://doi.org/10.3390/cancers15092435
[20] Van Dessel, L. F., van Riet, J., Smits, M., Zhu, Y., Hamberg, P., van der Heijden, M. S., Bergman, A. M., van Oort, I. M., de Wit, R., Voest, E. E., Steeghs, N., Yamaguchi, T. N., Livingstone, J., Boutros, P. C., Martens, J. W. M., Sleijfer, S., Cuppen, E., Zwart, W., van de Werken, H. J. G., Mehra, N., & Lolkema, M. P. (2019). The genomic landscape of metastatic castration-resistant prostate cancers reveals multiple distinct genotypes with potential clinical impact. Nature Communications, 10(1), 5251. https://doi.org/10.1038/s41467-019-13084-7
[21] Kregel, S., Malik, R., Asangani, I. A., Wilder-Romans, K., Rajendiran, T., Xiao, L., Vo, J. N., Soni, T., Cieslik, M., Fernadez-Salas, E., Zhou, B., Cao, X., Speers, C., Wang, S., & Chinnaiyan, A. M. (2019). Functional and Mechanistic Interrogation of BET Bromodomain Degraders for the Treatment of Metastatic Castration-resistant Prostate Cancer. Clinical Cancer Research, 25(13), 4038-4048. https://doi.org/10.1158/1078-0432.CCR-18-3776
[22] Mateo, J., Seed, G., Bertan, C., Rescigno, P., Dolling, D., Figueiredo, I., Miranda, S., Nava Rodrigues, D., Gurel, B., Clarke, M., Atkin, M., Chandler, R., Messina, C., Sumanasuriya, S., Bianchini, D., Barrero, M., Petermolo, A., Zafeiriou, Z., Fontes, M., Perez-Lopez, R., Tunariu, N., Fulton, B., Jones, R., McGovern, U., Ralph, C., Varughese, M., Parikh, O., Jain, S., Elliott, T., Sandhu, S., Porta, N., Hall, E., Yuan, W., Carreira, S., & de Bono, J. S. (2020). Genomics of lethal prostate cancer at diagnosis and castration resistance. Journal of Clinical Investigation, 130(4), 1743-1751. https://doi.org/10.1172/JCI132031
[23] Nóbrega, M., Cilião, H. L., Souza, M. F., Souza, M. R., Serpeloni, J. M., Fuganti, P. E., & Cólus, I. M. S. (2020). Association of polymorphisms of PTEN, AKT1, PI3K, AR, and AMACR genes in patients with prostate cancer. Genetics and Molecular Biology, 43(3), e20180329. https://doi.org/10.1590/1678-4685-GMB-2018-0329
[24] Li, Y., Yang, R., Henzler, C. M., Ho, Y., Passow, C., Auch, B., Carreira, S., Nava Rodrigues, D., Bertan, C., Hwang, T. H., Quigley, D. A., Dang, H. X., Morrissey, C., Fraser, M., Plymate, S. R., Maher, C. A., Feng, F. Y., de Bono, J. S., & Dehm, S. M. (2020). Diverse AR Gene Rearrangements Mediate Resistance to Androgen Receptor Inhibitors in Metastatic Prostate Cancer. Clinical Cancer Research, 26(8), 1965–1976. https://doi.org/10.1158/1078-0432.CCR-19-3023
[25] Lin, Y. W., Wen, Y. C., Chu, C. Y., Tung, M. C., Yang, Y. C., Hua, K. T., Pan, K. F., Hsiao, M., Lee, W. J., & Chien, M. H. (2020). Stabilization of ADAM9 by N-α-acetyltransferase 10 protein contributes to promoting progression of androgen-independent prostate cancer. Cell Death & Disease, 11(7), 591. https://doi.org/10.1038/s41419-020-02786-2
[26] Das, R., Sjöström, M., Shrestha, R., Yogodzinski, C., Egusa, E. A., Chesner, L. N., Chen, W. S., Chou, J., Dang, D. K., Swinderman, J. T., Ge, A., Hua, J. T., Kabir, S., Quigley, D. A., Small, E. J., Ashworth, A., Feng, F. Y., & Gilbert, L. A. (2021). An integrated functional and clinical genomics approach reveals genes driving aggressive metastatic prostate cancer. Nature Communications, 12(1), 4601. https://doi.org/10.1038/s41467-021-24919-7
[27] Zhang, X., Huang, Z., Wang, J., Ma, Z., Yang, J., Corey, E., Evans, C. P., Yu, A. M., & Chen, H. W. (2021). Targeting Feedforward Loops Formed by Nuclear Receptor RORγ and Kinase PBK in mCRPC with Hyperactive AR Signaling. Cancers (Basel), 13(7), 1672. https://doi.org/10.3390/cancers13071672
[28] Tan, W., Zheng, T., Wang, A., Roacho, J., Thao, S., Du, P., Jia, S., Yu, J., King, B. L., & Kohli, M. (2022). Dynamic changes in gene alterations during chemotherapy in metastatic castrate resistant prostate cancer. Scientific Reports, 12(1), 4672. https://doi.org/10.1038/s41598-022-08520-6
[29] Sandhu, H. S., Portman, K. L., Zhou, X., Zhao, J., Rialdi, A., Sfakianos, J. P., Guccione, E., Kyprianou, N., Zhang, B., & Mulholland, D. J. (2022). Dynamic plasticity of prostate cancer intermediate cells during androgen receptor-targeted therapy. Cell Reports, 40(4), 111123. https://doi.org/10.1016/j.celrep.2022.111123
[30] Yun, J. W., Lee, S., Ryu, D., Park, S., Park, W. Y., Joung, J. G., & Jeong, J. (2019). Biomarkers Associated with Tumor Heterogeneity in Prostate Cancer. Translational Oncology, 12(1), 43-48. https://doi.org/10.1016/j.tranon.2018.09.003
[31] Zhou, X., Yang, X., Sun, X., Xu, X., Li, X., Guo, Y., Wang, J., Li, X., Yao, L., Wang, H., & Shen, L. (2019). Effect of PTEN loss on metabolic reprogramming in prostate cancer cells. Oncology Letters, 17(3), 2856-2866. https://doi.org/10.3892/ol.2019.9932
[32] Mateo, J., Seed, G., Bertan, C., Rescigno, P., Dolling, D., Figueiredo, I., Miranda, S., Nava Rodrigues, D., Gurel, B., Clarke, M., Atkin, M., Chandler, R., Messina, C., Sumanasuriya, S., Bianchini, D., Barrero, M., Petermolo, A., Zafeiriou, Z., Fontes, M., Perez-Lopez, R., Tunariu, N., Fulton, B., Jones, R., McGovern, U., Ralph, C., Varughese, M., Parikh, O., Jain, S., Elliott, T., Sandhu, S., Porta, N., Hall, E., Yuan, W., Carreira, S., & de Bono, J. S. (2020). Genomics of lethal prostate cancer at diagnosis and castration resistance. Journal of Clinical Investigation, 130(4), 1743-1751. https://doi.org/10.1172/JCI132031
[33] Turnham, D. J., Bullock, N., Dass, M. S., Staffurth, J. N., & Pearson, H. B. (2020). The PTEN Conundrum: How to Target PTEN-Deficient Prostate Cancer. Cells, 9(11), 2342. https://doi.org/10.3390/cells9112342
[34] Compérat, E., Wasinger, G., Oszwald, A., Kain, R., Cancel-Tassin, G., & Cussenot, O. (2020). The Genetic Complexity of Prostate Cancer. Genes (Basel), 11(12), 1396. https://doi.org/10.3390/genes11121396
[35] Nóbrega, M., Cilião, H. L., Souza, M. F., Souza, M. R., Serpeloni, J. M., Fuganti, P. E., & Cólus, I. M. S. (2020). Association of polymorphisms of PTEN, AKT1, PI3K, AR, and AMACR genes in patients with prostate cancer. Genetic and Molecular Biology, 43(3), e20180329. https://doi.org/10.1590/1678-4685-GMB-2018-0329
[36] Imada, E. L., Sanchez, D. F., Dinalankara, W., Vidotto, T., Ebot, E. M., Tyekucheva, S., Franco, G. R., Mucci, L. A., Loda, M., Schaeffer, E. M., Lotan, T., & Marchionni, L. (2021). Transcriptional landscape of PTEN loss in primary prostate cancer. BMC Cancer, 21(1), 856. https://doi.org/10.1186/s12885-021-08593-y
[37] Mirzaei, S., Paskeh, M. D. A., Okina, E., Gholami, M. H., Hushmandi, K., Hashemi, M., Kalu, A., Zarrabi, A., Nabavi, N., Rabiee, N., Sharifi, E., Karimi-Maleh, H., Ashrafizadeh, M., Kumar, A. P., & Wang, Y. (2022). Molecular Landscape of LncRNAs in Prostate Cancer: A focus on pathways and therapeutic targets for intervention. Journal of Experimental & Clinical Cancer Research, 41(1), 214. https://doi.org/10.1186/s13046-022-02406-1
[38] Khan, M. M., Mohsen, M. T., Malik, M. Z., Bagabir, S. A., Alkhanani, M. F., Haque, S., Serajuddin, M., & Bharadwaj, M. (2022). Identification of Potential Key Genes in Prostate Cancer with Gene Expression, Pivotal Pathways and Regulatory Networks Analysis Using Integrated Bioinformatics Methods. Genes (Basel), 13(4), 655. https://doi.org/10.3390/genes13040655
[39] Feng, E., Rydzewski, N. R., Zhang, M., Lundberg, A., Bootsma, M., Helzer, K. T., Lang, J. M., Aggarwal, R., Small, E. J., Quigley, D. A., Sjöström, M., & Zhao, S. G. (2022). Intrinsic Molecular Subtypes of Metastatic Castration-Resistant Prostate Cancer. Clinical Cancer Research, 28(24), 5396-5404. https://doi.org/10.1158/1078-0432.CCR-22-2567
[40] Pan, J., Zhao, J., Ni, X., Gan, H., Wei, Y., Wu, J., Zhang, T., Wang, Q., Freedland, S. J., Wang, B., Song, S., Ye, D., Liu, C., & Zhu, Y. (2022). The prevalence and prognosis of next-generation therapeutic targets in metastatic castration-resistant prostate cancer. Molecular Oncology, 16(22), 4011-4022. https://doi.org/10.1002/1878-0261.13320
[41] Tan, W., Zheng, T., Wang, A., Roacho, J., Thao, S., Du, P., Jia, S., Yu, J., King, B. L., & Kohli, M. (2022). Dynamic changes in gene alterations during chemotherapy in metastatic castrate resistant prostate cancer. Scientific Reports, 12(1), 4672. https://doi.org/10.1038/s41598-022-08520-6
[42] Alzoubi, A., Al Bashir, S., Smairat, A., Alrawashdeh, A., Haddad, H., & Kheirallah, K. (2023). PTEN loss is not a determinant of time to castration-resistance following androgen-deprivation therapy in prostate cancer: A study from Jordan. Journal of Medical Life, 16(4), 593-598. https://doi.org/10.25122/jml-2023-0034